CFD & Thermal Analysis
1. Thermal Analysis
Thermal analysis is a set of techniques used in materials science and chemistry to study the physical and chemical properties of materials as they respond to changes in temperature. These techniques are valuable for characterizing a wide range of materials, including polymers, metals, ceramics, and composites, to gain insights into their behavior and properties under different thermal conditions. Some common methods of thermal analysis include:
i. Differential Scanning Calorimetry (DSC): DSC measures the heat flow into or out of a sample as it is heated or cooled, relative to a reference material. It is used to determine properties such as melting points, glass transition temperatures, heat capacity, and reaction enthalpies.
ii. Thermogravimetric Analysis (TGA): DMA measures the mechanical properties of a material (e.g., stiffness, elasticity, and damping) as a function of temperature or frequency. It is often used to study the viscoelastic behavior of polymers.
iii. Dynamic Mechanical Analysis (DMA): DSC measures the heat flow into or out of a sample as it is heated or cooled, relative to a reference material. It is used to determine properties such as melting points, glass transition temperatures, heat capacity, and reaction enthalpies.
iv. Thermal Gravimetric-Differential Scanning Calorimetry (TGA-DSC): This technique combines TGA and DSC to simultaneously measure weight loss and heat flow in a material, providing complementary information about thermal events and decomposition reactions.
v. Thermo-optical Analysis: This includes techniques like thermooptical analysis (TOA) and thermomechanical analysis (TMA), which study changes in the optical properties or dimensions of materials as a function of temperature.
These techniques provide valuable information about phase transitions, thermal stability, decomposition kinetics, and other thermal properties of materials. Researchers and industries use thermal analysis to develop and improve materials for various applications, such as manufacturing processes, product quality control, and the design of advanced materials in fields like electronics, aerospace, and pharmaceuticals.
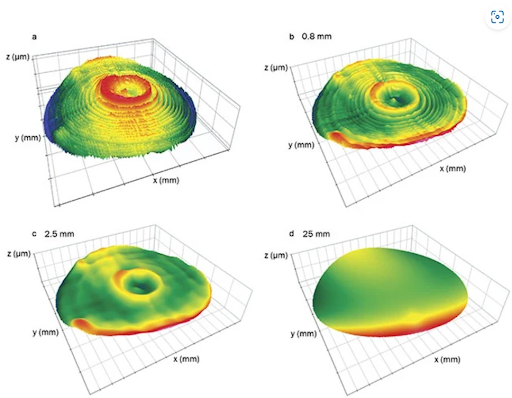
2. Rotating machinery
Rotating machinery refers to a broad category of mechanical systems and equipment that involve the rotation of components to perform various tasks and functions. These machines are commonly found in a wide range of industries and applications due to their ability to convert mechanical energy into useful work. Some common examples of rotating machinery include:
i. Electric Motors and Generators: Electric motors are used to convert electrical energy into mechanical energy by rotating a shaft, which can be used to drive various devices or machinery. Generators, on the other hand, convert mechanical energy (often from a rotating source) into electrical energy.
ii. Turbines: Turbines are machines that use the kinetic energy of a fluid (such as steam, water, or gas) to rotate a shaft and produce mechanical work or generate electricity. They are commonly used in power plants, aircraft engines, and propulsion systems.
iii. Pumps and Compressors: Pumps are devices that use rotating impellers to transfer liquids from one location to another, while compressors use rotating components to increase the pressure of gases. Both are vital in industries like manufacturing, agriculture, and HVAC (heating, ventilation, and air conditioning).
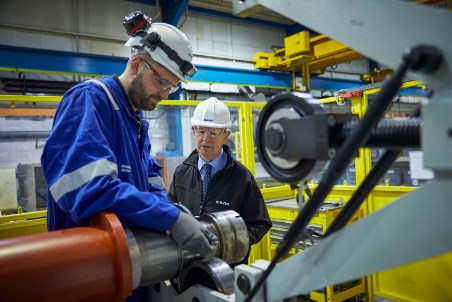
Fig: Improving performance in rotating machinery
iv. Rotary Engines: Rotary engines, such as the Wankel engine, have a rotating rotor that moves within a housing to convert combustion energy into mechanical motion.
v. Rotating Mixers and Agitators: These machines are used in chemical, pharmaceutical, and food processing industries to mix, blend, or agitate materials in tanks or containers.
vi. Rotating Drilling Equipment: Equipment like drilling rigs and drill bits use rotation to create holes in the ground or materials for purposes like oil and gas exploration, mining, and construction.
Effective operation, maintenance, and monitoring of rotating machinery are crucial in many industrial processes to ensure safety, efficiency, and reliability. This involves techniques such as vibration analysis, oil analysis, and condition monitoring to detect and address potential issues before they lead to equipment failure. The field of mechanical engineering plays a key role in the design, analysis, and optimization of rotating machinery to meet specific performance and reliability requirements.
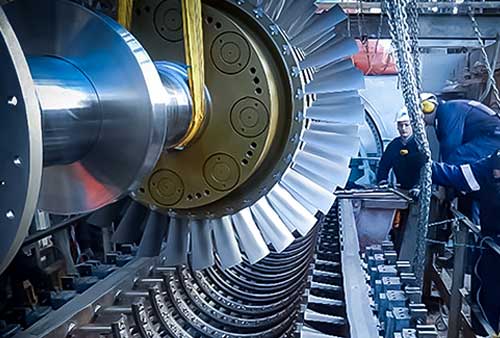
Fig: Rotating Machinery Services (RMS)
3. Fluid Structure Interaction
Fluid-structure interaction (FSI) is the interaction of
a fluid flow with a solid structure. It is a multiphysics problem that involves
the solution of the Navier-Stokes equations for the fluid flow and the equations
of structural mechanics for the solid structure. The two sets of equations are
coupled together through the boundary conditions, which enforce the continuity
of velocity and traction at the interface between the fluid and the structure.
FSI problems can be classified into two types:
- Steady-state FSI: In this type of problem, the fluid flow and the structural deformation are assumed to be time-invariant.
- Unsteady FSI: In this type of problem, the fluid flow and the structural deformation are allowed to vary with time.
FSI
problems are often encountered in many engineering applications,
such as:
The numerical simulation of FSI problems is a challenging task, and there are a number of different methods that can be used. The most common methods are the finite element method (FEM) and the boundary element method (BEM).
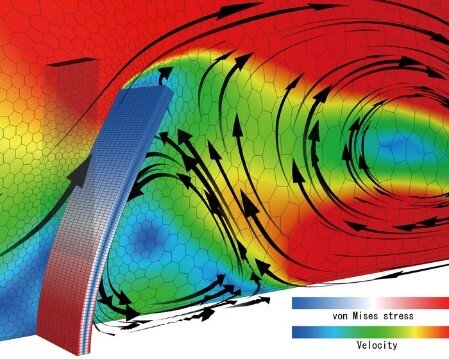
Fig: FLUID-STRUCTURE INTERACTION (FSI)
4. Multiphase flow analysis
Multiphase flow analysis is the study of the flow of two or more fluids or a fluid and a solid in the same region. It is a complex problem because the different fluids or phases can interact with each other in a variety of ways, depending on their properties and the flow conditions. There are a number of different methods that can be used to analyze multiphase flow, including:
- Computational fluid dynamics (CFD): CFD is a powerful numerical method that can be used to simulate the flow of fluids. CFD can be used to study a wide variety of multiphase flow problems, including bubble flow, droplet flow, and gas-liquid flow.
- Experimental methods: Experimental methods can be used to measure the properties of multiphase flows, such as the velocity, pressure, and temperature of the fluids. Experimental methods can also be used to visualize the flow of fluids.
- Analytical methods: Analytical methods can be used to solve simplified models of multiphase flow problems. Analytical methods are often used to gain a better understanding of the fundamental physics of multiphase flow.
FSI
Multiphase flow analysis is used in a wide variety of
engineering applications, including:
The numerical simulation of FSI problems is a challenging task, and there are a number of different methods that can be used. The most common methods are the finite element method (FEM) and the boundary element method (BEM).
Fig: Multiphase Fluid Flow Analysis
5.Aeroacoustic analysis
Aeroacoustic analysis is a specialized field of study within acoustics and fluid dynamics that focuses on understanding and predicting noise generated by the motion of air (or other gases) as it interacts with solid objects or other fluids. It is particularly relevant in the aerospace and automotive industries, where reducing noise emissions is a critical consideration for improving the comfort of passengers and reducing the environmental impact of vehicles. Here are some key aspects of aeroacoustic analysis:
i. Noise
Sources:
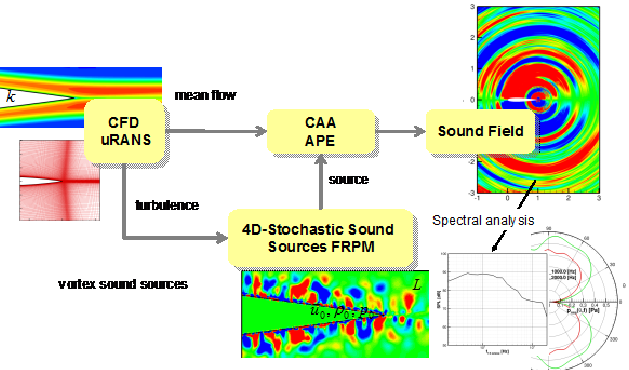
Fig: Aeroacoustic analysis procedure
ii.
Measurement and Analysis Techniques:
iii.
Applications:
6. Free surface analysis and optimization
Free surface analysis and optimization are techniques used in fluid dynamics and engineering to study and improve the behavior of fluid surfaces that are not confined by solid boundaries. This typically involves understanding and optimizing the shape, dynamics, and interactions of free surfaces, which can be encountered in various applications, including naval architecture, aerospace, civil engineering, and manufacturing. Here's an overview of free surface analysis and optimization:
Free Surface Analysis:
A free surface is the boundary or interface between a fluid (liquid or gas) and the surrounding environment, where the fluid is not constrained by solid walls or boundaries. Examples include the water-air interface in oceans, rivers, and tanks, as well as the fuel-air interface in aircraft fuel tanks.
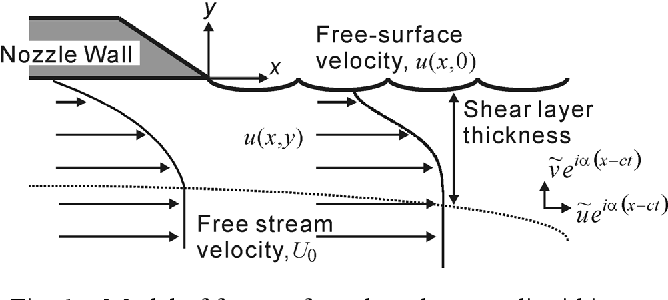
Fig: Free Surface analysis
Free Surface Optimization:
Free surface optimization aims to find the optimal shape or behavior of a free surface to achieve specific engineering goals. This can involve minimizing drag, maximizing stability, or improving efficiency, among other objectives.
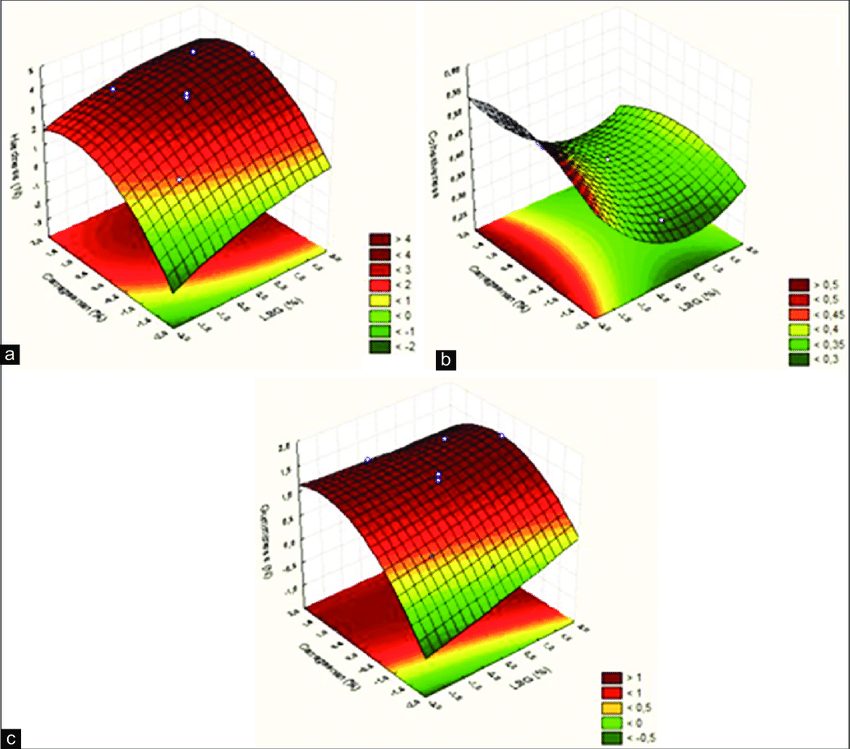
Fig: Surface Optimization
EF Analysis
1. Static Structural Analysis
Static structural analysis is a branch of structural engineering and finite element analysis (FEA) that focuses on evaluating the behavior of structures under static loading conditions. It is a crucial step in the design and assessment of various engineering structures, including buildings, bridges, aircraft, and mechanical components
2. Dynamic Analysis
Dynamic analysis is the process of testing and evaluating a program — while software is running. Also referred to as dynamic code scanning, dynamic analysis improves the diagnosis and correction of bugs, memory issues, and crashes of an application during its execution.
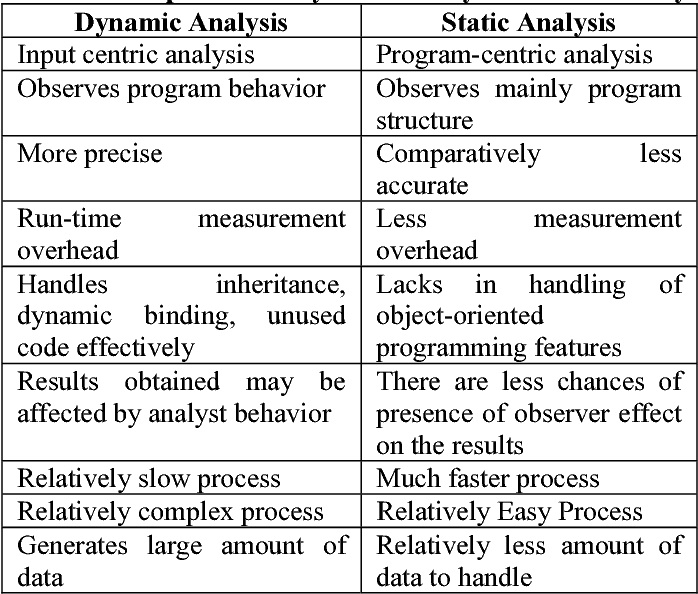
3. Kinematic analysis
Kinematic analysis is a fundamental concept in the field of mechanics and engineering, particularly in the study of motion. It involves the examination of the motion of objects, including their displacement, velocity, and acceleration, without considering the forces causing that motion.
4. Thermal Analysis
Thermal analysis is a set of techniques used in materials science and chemistry to study the physical and chemical properties of materials as they respond to changes in temperature. These techniques are valuable for characterizing a wide range of materials, including polymers, metals, ceramics, and composites, to gain insights into their behavior and properties under different thermal conditions.
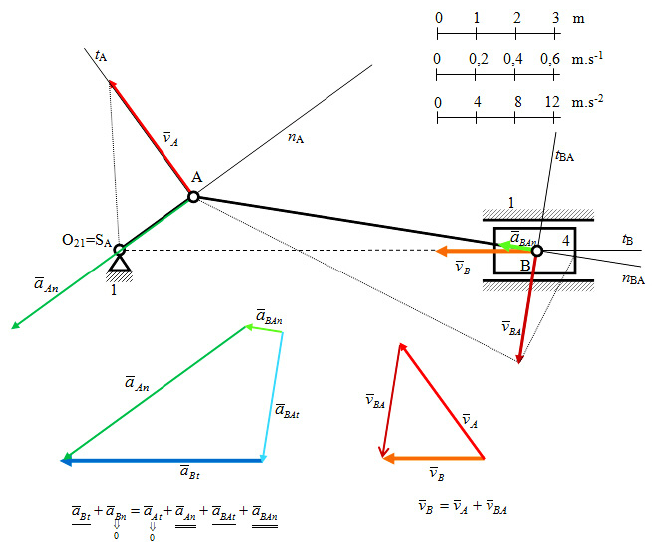
Fig: Kinematic analysis - graphical solution
5. Durability & Fatigue
Durability and fatigue are important concepts in various fields, including materials science, engineering, and mechanics. Here's some information about each of these terms:
Durability: Durability refers to the ability of a material, product, or structure to withstand wear, decay, or damage over an extended period of time. It is a critical factor in the design and evaluation of various products and systems. Durability testing involves subjecting materials to various environmental conditions, stressors, or usage scenarios to assess their long-term performance.
Fatigue: Fatigue, in the context of materials and structural engineering, is the progressive and localized structural damage that occurs when a material undergoes cyclic loading or repeated stress over time. This phenomenon is particularly relevant in situations where materials or components are subjected to fluctuating stress levels, such as bridges, aircraft, and mechanical components. Fatigue failure can lead to catastrophic consequences if not properly managed or accounted for in design.
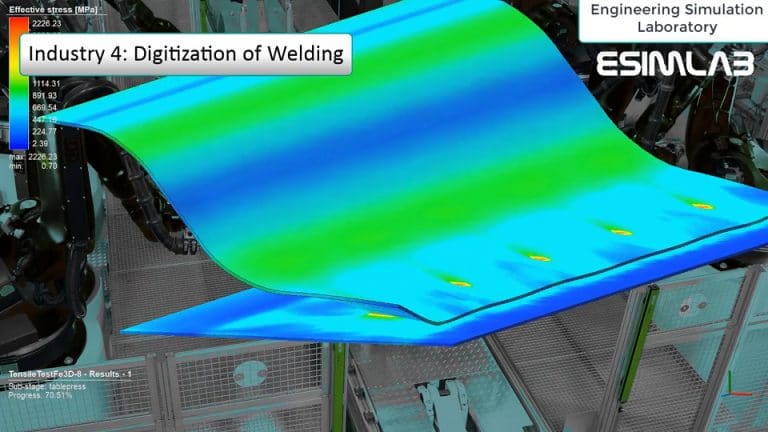
Fig: Durability and Fatigue Life Prediction with FEA Simulation
6. Optimization
Finite Element (FE) analysis optimization is the application of optimization techniques in the context of finite element analysis. It involves using computational methods to find the best possible design or configuration of a structure or system while considering various constraints and objectives. FE analysis optimization is commonly used in engineering and design processes to improve the performance, efficiency, or cost-effectiveness of products and systems.